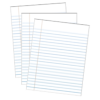
Infrared radiation
Storyboard
Infrared radiation corresponds mainly to the energy radiated by the earth. A small part of it is irradiated directly into space while the vast majority is absorbed by clouds. These in turn radiate both returned to earth and space. The origin of global warming is mainly a consequence of this flow from the earth to the atmosphere and from the latter to the surface of the earth.
ID:(536, 0)
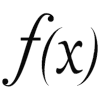
Emission NIR intensity from the earth's surface to space
Equation
Just like in visible radiation, the atmosphere interacts with infrared radiation, which is mostly
$\lambda > 750\,nm$ |
Similar to the situation with visible radiation, where the interaction with the atmosphere was modeled using the visible coverage _v, the fraction _i can be introduced to describe its effect on infrared radiation. Also in this case, the radiation that doesn't interact can be calculated using
$ I_p =(1- \gamma ) I_s $ |
Thus, the emitted radiation from the Earth I_e that doesn't interact exits the planet into space. This is
![]() |
ID:(4677, 0)
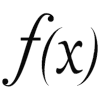
Emission intensity NIR from the earth to the atmosphere
Equation
From the terrestrial radiation $I_e$, which mostly
$\lambda > 750\,nm$ |
The fraction of radiation that interacts with the atmosphere is calculated using the coverage $\gamma$ through
$ I_p = \gamma I_s $ |
In the case of radiation emitted by the Earth's surface, a fraction $\gamma_i$ interacts with the atmosphere, generating a flux
![]() |
ID:(4684, 0)
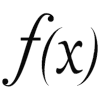
Emission intensity NIR from the earth's surface
Equation
If the Earth is at a temperature $T_e$, it emits radiation according to the Stefan-Boltzmann law with an intensity given by the following formula:
![]() |
If the Earth is at a temperature $T_s$, it emits radiation, mainly at wavelengths $\lambda > 750$ nm, with a power given by the Stefan-Boltzmann law:
$ P = \sigma \epsilon S T_s ^4$ |
where $\sigma$ is the Stefan-Boltzmann constant, $\epsilon$ is the emissivity, and $S$ is the emitting surface area.
The intensity of the radiation is defined as the power per unit area, so we can express it as:
$ I =\displaystyle\frac{ P }{ S }$ |
where $S$ is the emitting area.
Thus, the intensity emitted from the Earth's surface $I_e$ is given by:
$ I_e = \sigma \epsilon T_e ^4$ |
where $T_e$ is the temperature and $\epsilon$ is the emissivity of the surface.
Where $\sigma$ is the Stefan-Boltzmann constant and $\epsilon$ is the emissivity coefficient. The Stefan-Boltzmann constant $\sigma$ has a value of approximately $5.67 \times 10^{-8} W/m^2K^4$, and the emissivity coefficient $\epsilon$ represents the efficiency with which the Earth's surface emits radiation, ranging from 0 to 1.
ID:(4676, 0)
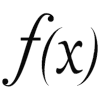
Emisión infrarroja de la parte inferior de la Atmosfera
Equation
The intensity $I$ emitted by a body at temperature $T$ is governed by the Stefan-Boltzmann law, which is expressed as:
$ I = \sigma \epsilon S T ^4$ |
where $\epsilon$ is the emissivity and $\sigma$ is the Stefan-Boltzmann constant. Therefore, in the case of the lower edge of the cloud, which has a temperature $T_b$, the intensity will be:
![]() |
ID:(4679, 0)
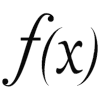
Emisión infrarroja de la parte superior de la Atmosfera
Equation
Si la parte superior de la atmósfera esta a una temperatura
$\lambda > 750\,nm$ |
según a la ley de Stefan Boltzmann con
$ P = \sigma \epsilon S T_s ^4$ |
que en este caso resulta con
$ I_t = \sigma \epsilon T_t ^4$ |
donde
ID:(4680, 0)

Distribution of heat transported by convection
Description
If we observe the distribution of heat transported by convection over the planet's surface, it can be noticed that there are more or less constant levels. On one hand, we have oceanic and continental zones with a flux around $17 W/m^2$ (upward) and approximately $-30 W/m^2$ (downward) in areas covered with snow and ice:
None
These data are derived from a 40-year reanalysis conducted by Kallberg P., Berrisford P., Hoskins B., Simmons A., Uppala S., Lamy-Thepaut S., Hine R., 2005: ERA-40 Atlas. Reading, UK, ECMWF Re-Analysis Project (Kallberg et al., 2005).
ID:(9263, 0)
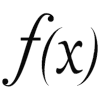
Conduction and evaporative flow
Equation
By modeling conduction and evaporation transport, a relationship can be established for heat transport as follows:
where the constants are defined as , and their values are on the order of $\kappa_l\sim 10.0 W/m^2$, $\kappa_c\sim 0.16 W/m^2K$, and a velocity of $8 m/s$.
The term $\kappa_l$ mainly arises from the energy transported by the movement of moist air masses that release energy upon condensation. The term $\kappa_c$ originates from air transport through convection and the corresponding adiabatic expansion, thus depending primarily on the temperature gradient:
![]() |
However, it is important to note that this is a simplification.
ID:(9270, 0)

Emisión onda larga de la tierra en función del tiempo (D0+1)
Php
Si se observa la radiación de onda larga (NIR) se ve que existe un máximo en torno al mes de agosto/septiembre de todos los años:
Esto se debe a que el hemisferio norte presenta mayor masas continentales por lo que estas reflejan mayormente cuando es verano en dicho hemisferio..
ID:(9324, 0)

Emisión onda larga de la tierra en función de la latitud (D1+0)
Php
La radiación de onda larga (NIR) es en primera aproximación simétrica en torno al ecuador fuera de presentar un máximo en torno de los grados -20 y +20:
Esto corresponde tanto a la falta de masa continental en torno al ecuador y la baja de intensidad hacia los polos por efecto de la incidencia inclinada de la radiación.
ID:(9325, 0)