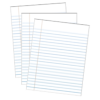
What is physics?
Storyboard 
Physics, in its beginnings, emerged as a branch of science rooted in philosophical thought, aiming to develop objective models based on mathematical equations. These models sought not only to describe physical systems but also to empirically validate their accuracy. This approach culminated in the formulation of the scientific method, which came to encompass all non-living systems, from the subatomic to the cosmological scale.
In a later phase, physics expanded into areas involving more complex processes across nearly all natural sciences, successfully explaining phenomena that had previously defied understanding. Today, physics is applied to fields as diverse as Earth sciences and materials science. Furthermore, it has ventured into the biological and medical fields, providing models that help explain a wide range of processes and enabling informed interventions supported by a vast array of empirically verified models.
The advancement continues, and the current frontiers of physics include topics involving human behavior, such as sociology and economics. Some physicists and mathematicians have even applied their tools to financial markets, achieving significant wealth by accurately predicting risk rates and understanding how market participants act, thus effectively intervening for personal benefit.
Ultimately, the key is to view physics as a tool for understanding how any system operates, building models, using them to analyze potential situations, and accurately predicting how those systems will behave.
ID:(2120, 0)
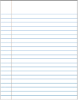
Traditional definition
Description 
Before applying the newly introduced concepts, let's establish a common understanding of what physics is.
During the Scientific Revolution, key figures like Galileo Galilei, Isaac Newton, and René Descartes helped to separate physics from natural philosophy, allowing it to consolidate as an independent discipline. This new science used mathematical models to describe phenomena such as motion, optics, and mechanics. In his work Principia Mathematica (1687) [1], Newton presented a vision in which natural phenomena could be explained through universal mathematical laws, establishing a solid mathematical and empirical foundation for physics.
Galileo Galilei [2], Isaac Newton [3], René Descartes [4]
Over time, the study of phenomena such as electricity, magnetism, and thermodynamics expanded the fields within physics and strengthened the experimental approach. The contributions of Michael Faraday, James Clerk Maxwell, and Ludwig Boltzmann promoted a more quantitative physics, in which matter and energy were understood through universal laws and experimental methods, solidifying physics as a science based on measurable and reproducible principles.
Michael Faraday [5], James Clerk Maxwell [6], Ludwig Boltzmann [7]
In the 20th century, modern physics, understood as the study of matter and energy at all scales, was consolidated with the development of quantum mechanics and the theory of relativity. Figures like Albert Einstein, Niels Bohr, and Werner Heisenberg expanded the scope of physics to include phenomena on the subatomic scale, while Edwin Hubble extended its application to the cosmos and galaxies.
Albert Einstein [8], Niels Bohr [9], Werner Heisenberg [10], Erwin Hubble [11]
Thus, physics tends to be defined as the science that uses mathematical models and experiments to explain the properties and behavior of matter and energy, covering phenomena from the subatomic to the galactic level.
However, while this definition describes the vast scope of physics, it does not delve into its methodological approach, how it operates, or the types of results it can achieve.
Books
[1] Principia Mathematica (Mathematical Principles), Isaac Newton (1687)
Images
[2] Justus Sustermans, Public domain, via Wikimedia Commons
[3] James Thronill after Sir Godfrey Kneller, Public domain, via Wikimedia Commons
[4] After Frans Hals, Public domain, via Wikimedia Commons
[5] Thomas Phillips, Public domain, via Wikimedia Commons
[6] George J. Stodart, Public domain, via Wikimedia Commons
[7] Universität Wien, Public domain, via Wikimedia Commons
[8] Bettmann / Contributor via Getty Images
[9] The American Institute of Physics credits the photo [1] to AB Lagrelius & Westphal, which is the Swedish company used by the Nobel Foundation for most photos of its book series Les Prix Nobel., Public domain, via Wikimedia Commons
[10] Bundesarchiv, Bild 183-R57262 / Unknown author / CC-BY-SA 3.0
[11] Johan Hagemeyer, Public domain, via Wikimedia Commons
ID:(15900, 0)
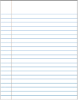
How physics works
Description 
The essence of physics lies in the fact that, as a science, it uses the scientific method to develop hypotheses that lead to models explaining how the world around us functions. It is not limited to a superficial description but allows precise estimations of the values of the parameters involved, relying on universal constants, specific constants, and mathematical relationships ideally derived from fundamental principles. These evaluations enable the rigorous application of the scientific method, as the calculated values can be measured and verified, thereby confirming the model's accuracy relative to reality.
Francis Bacon [4], René Descartes [5], Karl Popper [6]
The foundation of the modern scientific method is based on the work of Francis Bacon [1], who proposed an inductive approach, emphasizing the importance of observation and experimentation. This approach was complemented by René Descartes' [2] systematic and deductive method. Finally, the concept of falsifiability in hypotheses, introduced by Karl Popper [3], completed this framework, establishing that a scientific theory must be refutable to be considered valid.
In summary, the scientific method involves studying a physical system through the following steps:
1. Observing and characterizing reality.
2. Formulating a hypothesis about how the system functions.
3. Developing a model and making predictions based on the hypothesis.
4. Empirically verifying whether the predictions match reality.
The last step is repeated until a significant discrepancy is found between the predictions and the observed reality. In such a case, it is necessary to modify the hypothesis to ensure it adequately explains reality, or, if that is not possible, to discard it altogether.
Books
[1] Novum Organum Scientiarum (New Instrument of Science), Francis Bacon (1620)
[2] Discours de la méthode (Discourse on the Method), René Descartes (1637)
[3] The Logic of Scientific Discovery, Karl Popper (1934)
Images
[4] National Portrait Gallery, Public domain, via Wikimedia Commons
[5] After Frans Hals, Public domain, via Wikimedia Commons
[6] Lucinda Douglas-Menzies link, No restrictions, via Wikimedia Commons
ID:(15901, 0)
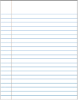
Other areas supported by physics
Description 
Today, the scientific method in physics is applied not only in traditional areas, covering everything from the subatomic world to galaxies, but also in non-traditional areas that have adopted the principles and methods of physics to address their specific challenges.
Traditional Areas of Physics
Physics continues to advance in traditional fields such as particle physics (which studies subatomic particles and fundamental forces), nuclear physics, astrophysics, and cosmology (which explore the universe on a grand scale). It also remains active in foundational areas like mechanics, electromagnetism, optics, thermodynamics, and condensed matter physics.
Non-Traditional Areas of Physics
In recent years, physics has expanded its influence into disciplines once considered outside its scope, applying its principles to analyze complex and varied problems. This phenomenon has led to the development of what is sometimes called "topic-specific physics," where physical models are used to study phenomena in different disciplines. Examples of these areas include:
Physics Applied to Health Sciences
Physics in Dentistry: Analyzes the mechanics of dental materials, chewing forces, and tissue behavior, improving prosthetics and orthodontic treatments.
Physics in Medicine: Applies physical principles in diagnostics and treatments, such as MRI, tomography, and radiotherapy.
Physics in Kinesiology: Studies human biomechanics, essential for rehabilitation and therapy development.
Neurophysics: Explores the nervous system through physics, investigating neural signal transmission and electrical processing.
Physics in Earth and Environmental Sciences
Ocean Physics: Investigates ocean dynamics, including currents and tides, vital for studying climate and marine ecosystems.
Soil Physics: Studies soil properties like permeability and structure, essential in agriculture and conservation.
Climate Physics: Analyzes physical processes governing Earth's climate, improving predictions and modeling climate change.
Environmental Physics: Examines the environment through a physical perspective, including contaminant dispersion and solar impact on ecosystems.
Geophysics: Applies physical methods to study Earth's structure, exploring seismology, magnetism, and plate tectonics.
Physics in Renewable Energy: Studies the conversion and efficiency of clean energy sources, like solar, wind, and geothermal, using principles of thermodynamics and materials physics.
Physics in Biological Sciences and Biotechnology
Biophysics: Applies physical principles to biological processes, addressing protein structures, ion flow, and DNA mechanics.
Astrobiology and Space Life Physics: Explores the possibility of life on other planets and how organisms adapt to extreme space conditions.
Nanotechnology: Studies material properties at the nanoscale, developing applications in medicine, electronics, and advanced materials.
Physics in Material Sciences and Advanced Technologies
Materials Physics: Studies mechanical, electrical, and optical properties of materials, essential for developing semiconductors and superconductors.
Physical Chemistry: Combines physics and chemistry to investigate chemical reactions and molecular properties through thermodynamics and kinetics.
Agrophysics: Applies physical principles to agriculture, analyzing soil structure, nutrient dynamics, and heat transfer in crops.
Physics in Renewable Energy: Uses physics to improve efficiency and utilization of clean energy sources.
Physics in Social Sciences and Humanities
Psychophysics: Examines the relationship between physical stimuli and sensory perceptions, studying how the brain interprets stimuli like light and sound.
Sociophysics: Applies statistical physics methods to analyze social phenomena, like opinion spread and collective behavior.
Econophysics: Uses physical theories, especially statistics, to model economic systems and financial phenomena.
Urban Physics and Transportation: Models traffic flow and optimizes urban transportation using statistical physics and fluid dynamics.
Physics in Computational Sciences and Simulation
Computational Physics: Uses simulations and mathematical models to solve complex problems, such as climate modeling and particle simulation.
Physics in Artificial Intelligence (AI) and Machine Learning: Applies computational physics and statistics to optimize AI algorithms and neural networks.
Physics in Forensic Sciences and Conservation
Forensic Physics: Uses physical techniques to solve criminal investigations, such as ballistics, accident reconstruction, and isotopic dating.
Art Conservation Physics: Applies physical techniques, like spectroscopy, for the conservation and analysis of artworks and ancient materials.
Physics in Information Sciences and Cryptography
Physics of Complex Systems and Chaos Theory: Studies nonlinear systems and unpredictable behaviors in areas like meteorology, ecology, and economics.
Network Physics: Analyzes complex networks, like social, biological, and transportation networks, using statistical physics to understand their structure and dynamics.
Information Science and Quantum Cryptography: Explores quantum principles to develop secure communications and quantum information transmission.
Impact of Physics Expansion
This expansion of physics into new applications demonstrates its versatility and relevance, as it provides tools and models capable of analyzing and understanding complex phenomena across a wide spectrum of disciplines, from natural sciences and medicine to social sciences and economics. By adopting physical approaches, these areas benefit from greater precision in their methods and a deeper understanding of the phenomena they study, significantly contributing to scientific and technological advancement.
ID:(15903, 0)
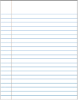
The Future of Physics Work Methodologies
Description 
It is increasingly evident that physicists and mathematicians are venturing into diverse areas far beyond the traditional inanimate world of classical physics. Simultaneously, in these new fields, the degree of modeling and mathematical formulation is growing more sophisticated to understand systems that are often no longer restricted to the non-human domain. With the advent of artificial intelligence systems, we are now witnessing the emergence of data-driven models that achieve high levels of accuracy, even if the underlying mathematics is not fully understood.
The common thread in this evolution remains hypothesis-based work, including modeling, making predictions, and empirical validation. While current modeling practices are rooted in mathematics, it is anticipated that new forms of modeling will emerge with the development of artificial intelligence. In this regard, the technique presented here, based on networks of equations, could naturally transition to data-driven networks. However, it is also possible that we will see a dramatic rise in phenomenological models based on artificial intelligence, which can achieve high precision but do not necessarily explain why a system behaves as it does.
For all these reasons, this tool should be seen not only as a support system for understanding and applying traditional physics but also as a pathway for transitioning to new approaches. This will help address potentially radical changes that we may not yet fully envision.
ID:(15902, 0)