
Amount of water vapor
Concept 
When the volume variation in phase change ($\Delta V$) changes phase from a liquid to a gas, it can be expressed as:
$\Delta V = V_{\text{gas}} - V_{\text{liquid}}$
Since the volume of the gas is significantly greater than that of the liquid,
$V_{\text{gas}} \gg V_{\text{liquid}}$
we can approximate:
$\Delta V \approx V_{\text{gas}}$
Given that water vapor behaves similarly to an ideal gas, we can state that with the values of the universal gas constant ($R$), the number of moles ($n$), the absolute temperature ($T$), and the water vapor pressure unsaturated ($p_v$):
Therefore, the volume variation in phase change ($\Delta V$) is:
$\Delta V = \displaystyle\frac{nRT}{p_v}$
ID:(3185, 0)
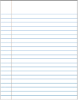
Condensation
Description 
Whenever the concentration of water vapor exceeds the saturated concentration the molecules that reach a surface tend to be captured by it. A thin layer of water begins to form and the phenomenon is called condensation. This occurs especially in cold objects since in the vicinity of these surfaces the air is colder and therefore tends to have a lower saturation concentration than in the rest of the space.
ID:(123, 0)
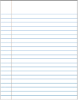
Water Vapor Pressure
Description 
By studying how water phase changes occur we can understand how this evaporates. With the gas equations, the evaporated amount can finally be estimated and with it the pressure generated by the water vapor. This pressure is the key to understanding how the gas travels to and from the ground to the environment.
ID:(1013, 0)

Relative humidity, concentration
Equation 
The relationship between the concentration of water vapor molecules ($c_v$) and saturated water vapor concentration ($c_s$) is referred to as the relative humidity ($RH$). In other words, when a relative humidity of 100% is reached, the existing concentration will be equal to the saturated concentration.
![]() |
ID:(3175, 0)

Pressure saturated water vapor
Equation 
The pressure saturated water vapor ($p_s$) can be calculated using the reference pressure ($p_{ref}$), the universal gas constant ($R$), the absolute temperature ($T$) and the molar Latent Heat ($l_m$) according to the following formula:
![]() |
Using the Clausius-Clapeyron equation for the gradient of the pressure ($p$) with respect to the absolute temperature ($T$), which depends on the latent Heat ($L$) and the volume variation in phase change ($\Delta V$):
$\displaystyle\frac{ dp }{ dT }=\displaystyle\frac{ L }{ \Delta V T }$ |
In the case of the phase change from liquid to gas, we can assume that the change in volume is approximately equal to the volume of the vapor. Therefore, we can employ the ideal gas equation with the number of moles ($n$), the volume ($V$), the universal gas constant ($R$), and the water vapor pressure unsaturated ($p_v$):
$$ |
Since the Clausius-Clapeyron equation can be written as:
$\displaystyle\frac{dp}{dT}=\displaystyle\frac{L}{n}\displaystyle\frac{p}{R T^2}$
Where the molar Latent Heat ($l_m$) ($l_m = L/n$) corresponds to the change in enthalpy during the phase change h (the energy required to form water), we finally have:
$\displaystyle\frac{dp}{dT}=l_m\displaystyle\frac{p}{RT^2}$
If we integrate this equation between the pressure saturated water vapor ($p_s$) and the pressure at point
$p_s=p_0e^{l_m/RT_0}e^{-l_m/RT}$
If we evaluate this expression with the data at the critical point:
$p_{ref}=p_0e^{l_m/RT_0}$
We finally have:
$ p_s = p_{ref} e^{- l_m / R T }$ |
ID:(3182, 0)